CRISPR, CRISPR, CRISPR….
Why is everyone talking about CRISPR and where is it taking us?
author: Kevin Curran PhD
updated: 11-6-2020
CRISPR is a new tool that allows scientists to edit DNA. Everyone is talking about CRISPR because this tool is more efficient than previous DNA editing techniques.
Editing DNA is nothing new. Biologists have been doing this for decades. However, CRISPR has made editing much cheaper and much easier from a logistical standpoint.
To illustrate this point…I recently attended a community event at my local San Diego public library. I watched a group of high-school students learn to design CRISPR edits on their laptops. Within 2 hours, the students had it figured out.
Since 2012, we’ve seen a rapid increase in the number of research laboratories altering DNA with the CRISPR technique.
As I describe later, this technique can be used to add or remove a gene from human cells. If CRISPR lives up to the hype, genetic diseases will be systematically eliminated.
In this article, I will explain some of the key aspects of CRISPR. You can click on a topic below or scroll down and read the entire article.
The CRISPR world is moving fast. I will update this page every 6 months in an attempt to keep up with the field.
In theory, gene editing (CRISPR) could be used to create lifestyle enhancements, such as increased intelligence or athleticism. Is that likely to happen?
If those changes could happen, would they become heritable and lead to an optimized or master race?
In the adjacent video, I attempt to shed light on these complex questions. Subscribe to my YouTube channel to see similar videos.
~Kevin Curran
CRISPR can direct DNA edits to the human genome.
This is a dynamic, biological process with many moving parts.
In my opinion, this type of dynamic activity is best understood with a video.
First watch the movie, then I’ll further discuss key aspects to CRISPR below…
Let Jennifer Doudna explain CRISPR in this video.
Jennifer is a professor at UC Berkeley. She is widely regarded as the main inventor of CRISPR.
In this TED talk, Jennifer does a great job explaining the basics of this gene editing tool.
Hit the subscribe button below to follow Kevin’s newsletter on Substack.
Kevin Curran PhD is the founder of Rising Tide Biology. His newsletter explores exciting new developments in biotech, including: Artificial Intelligence in drug discovery and the use of gene editing to eliminate genetic disorders.
He also examines the traditional market structure of the pharmaceutical industry and provides ideas for alternative models that could enhance global patient access to new medicines.
CRISPR is a system used to modify human DNA.
The movie below is the first video capture of CRISPR cutting into DNA. At the end of the short video, you can see double stranded DNA break apart.
The video is grainy and dark, but it provides an astonishing first look at CRISPR in action.
A team from Kanazawa University and University of Tokyo in Japan used a technique called high-speed atomic-force microscopy to capture the shape of Cas9, the enzyme that actually does the DNA cutting.
Movie originally published in the following Nature Communications manuscript.
Real-space and real-time dynamics of CRISPR-Cas9 visualized by high-speed atomic force microscopy
(Shibata, 2017)
Next, let’s discuss why CRISPR is disruptive
Why is CRISPR significantly better than previous genome editing techniques?
The main innovation with CRISPR is the ability to create precise DNA edits using guide RNA.
The process of designing a customized guide RNA to direct these DNA edits is much easier than designing a customized protein to direct DNA edits.
Previous DNA editing techniques required the use of engineered proteins to direct DNA edits.
Have you heard of Zinc Finger Nuclease or TALENS? These are the previous techniques that scientists used to orchestrate an edit in DNA. Most non-scientists have not heard of these techniques. They are trickier than CRISPR because they require engineered proteins in order to work properly. This means you need a trained technician who is skilled in molecular biology to alter the shape of a protein called a transcription factor. The hope is that this engineered transcription factor protein will then attach to your gene of interest and initiate a DNA edit (a double stranded break in the DNA.)
This can be a challenge. First of all, it is challenging to properly engineer a protein via recombinant DNA technology. Then, once you have your modified protein, there is no guarantee it will bind and cut DNA at the correct location.
In contrast, when performing CRISPR, you only need to design a specific genetic sequence on a guide RNA strand.
This is dramatically easier than re-designing a protein.
How CRISPR lets you edit DNA
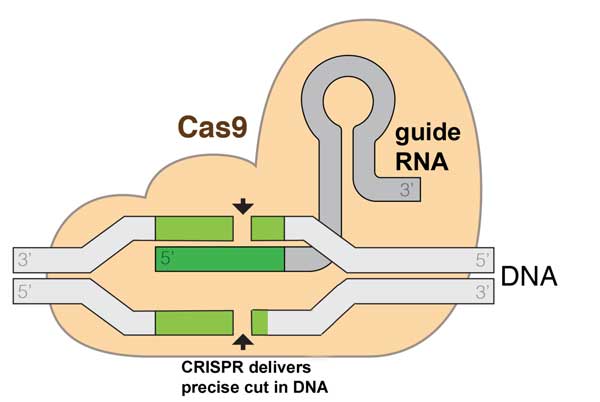
image: Marius Walter, CC BY-SA 4.0, via Wikimedia Commons
The CRISPR gene editing system consists of guide RNA and a CAS-9 protein.
The guide RNA directs the location of the CAS-9 cut. CAS-9 protein then cuts the DNA.
Once the DNA is cut, you have the option of mutating DNA or inserting DNA at the location of the cut.
Mutating the DNA can alter your gene of interest.
Inserting DNA can repair your gene of interest.
As I said earlier, I watched high school students learn to design guide RNA in two hours. You also have the option of outsourcing your guide RNA design. Many companies will be happy to design and construct your desired guide RNA strand. Then, for 20$, you can have your CRISPR tools sent to your home or workspace. In theory, your custom guide RNA could arrive by mail in a few days.
The guide RNA and CAS-9 protein will be shipped as genetic sequence on a circular structure called a plasmid. Once you’re ready, you insert your CRISPR plasmid into your cells. The CRISPR plasmid contains your guide RNA strand and sequence for CAS-9 protein. Once inside the cells, the guide RNA attaches to your gene of interest. Assuming your guide RNA design strategy is correct, then there is a high likelihood that this fragment of guide RNA will correctly bind at the intended location. The CAS-9 protein will then chop the DNA where the guide RNA is attached.
Ok, now that you have the general idea of CRISPR, let’s get into a bit more detail.
In this next video, Jennifer describes what happens after CRISPR directs a double stranded break in DNA.
In summary, there are two possible CRISPR options:
- If you want to disrupt a gene, you can rely on the cell’s natural system to repair the double strand break. This repair mechanism will often introduce a mutation in your gene of interest. This is helpful if you want to alter or remove the gene.
- If you want to repair a mutated gene, you can insert repair template with the CRISPR tools. This repair template is a chance for scientists to introduce new sections of DNA. The new DNA will insert at the location of the CAS-9 cut.
Where did this CRISPR system come from? Who gets credit?
Scientists discovered this gene editing system through their work with bacteria. Bacteria use CRISPR as a natural mechanism to help defend against viruses. Part of being a bacteria means you are often under assault from viruses. CRISPR is a cluster of DNA on the bacterial genome that can be deployed when a virus invades a bacteria. This DNA region will activate and join forces with a CAS-9 protein. In doing so – the bacteria will slice up the viral DNA before it infects the bacterial genome.
In summary, CRISPR evolved naturally within bacteria to help the bacteria fight viruses.
Did one person discover CRISPR?
Scientific discoveries usually occur because of many small contributions from lots of people. CRISPR is no different.
Many scientists observed that CRISPR is a natural defense system in bacteria. In 1993, Francisco Mojica was the first researcher to characterize what is now called CRISPR. However, Professor Doudna and her colleagues are credited with discovering how CRISPR can be used to direct genome edits in bacteria. These scientists modified the system to make it more user-friendly for other scientists. In 2012, the Doudna and Charpentier labs simplified the guide RNA component (combining 2 RNA elements into 1). They then confirmed that CRISPR could be used to direct double stranded DNA cuts at any desired location. In 2013, Dr. Feng Zhang (Broad Institute of MIT and Harvard) is credited with the key innovation of adjusting CRISPR so that it can direct DNA edits to mammalian cells (i.e. humans).
As you would imagine, there is currently a protracted legal battle occurring for the patent rights for CRISPR.
2019 update: A federal appeals court has, once again, ruled in favor of the Broad Institute. This ruling confirms an earlier US patent board decision, which states that Feng Zhang’s work did not “interfere” with patents at UC Berkeley. The Broad issued a statement reiterating their successful stance.
[our patents] are for genome editing in eukaryotic (including animal, human, and plant) cells, while the claims in UCB’s application were based on studies in cell-free systems and not directed to genome editing in eukaryotic cells.
2020 update:
The Broad Institute has secured its CRISPR patents in the United States, however the European Patent Office revoked one of its key patents in 2018. A February 2020 decision from the Board of Appeals at the European Patent Office has now revoked another Broad Institute claim to general patents on this technology. This recent move further strengthens the position of UC Berkeley in Europe.
Ok . . . CRISPR is a relatively cheap, easy and precise technique for genome modification.
So . . . how do we apply CRISPR to humans?
CRISPR is a tool that allows DNA editing. Once CRISPR is inside a cell, the guide RNA and CAS-9 can enter the nucleus, find the right location on the genome and initiate an edit. But, to be an effective therapy tool, we first need to deliver CRISPR to the correct cells in the human body. How is that going to work?
There are 2 different approaches to CRISPR delivery, in vivo and in vitro.
Keep in mind, this is a brand new field of science. Scientists are still working out details on the methods described below.
In vitro delivery
If technicians can remove the desired cells from the human body, then it is possible to directly deliver CRISPR exclusively to these cells. Living human cells can be kept alive in a dish. CRISPR can be applied to these cells, then – once modified – the cells can be returned back into the human body. This is called in vitro delivery.
For example, our blood cells (red blood cells, B cells, T cells) can be removed from our blood with a syringe. The blood cells can be kept alive in a dish while the CRISPR plasmid is added to the dish. A small burst of electricity (electroporation) is applied to the dish. This flash of electricity can help punch CRISPR across cell membranes and into the nucleus. These edited blood cells would then be transfused back into the patient.
In vivo delivery
What if we want to modify DNA from cells that cannot be easily removed from a sick patient?
Let’s say you are a patient suffering from a genetic disease that affects the retina cells in your eyes. Your retina cells contain a bad gene. This bad gene is making you blind. You only want CRISPR to remove this bad gene from your retina cells. You don’t want the other cells in your body to be affected.
How could we specifically target retina cells with CRISPR?
In this example, the CRISPR components (guide RNA and CAS-9) can be placed directly on your retina cells without the use of a virus or other complex delivery system. An eye dropper could be used to place a saline solution containing a CRISPR plasmid on the surface of your eyes. Then, perhaps a small (and safe) blast of electricity could help punch CRISPR across the retina cell membranes. If all of this goes smoothly, then CRISPR could direct a mutation in the middle of this bad gene…and you’re no longer blind.
Here’s the problem, only some types of cells in our body can be treated in vivo.
Cells in the eye, skin, muscle or liver should be relatively easy to access within the human body. The key word here is relatively. CRISPR in vivo delivery is currently a major challenge. Many companies are attempting to resolve this challenge.
Most cells in our body are not easy to access in vivo. Most cells in our body are either hard-to-reach or hard-to-infect. In this case, a virus may be necessary to deliver CRISPR.
Stem cells and neurons are examples of cells that often require a viral delivery system. This virus would need to be safe and designed so that it only infects specific cell types. There are other non-viral, in vivo delivery systems that are currently being explored: exosomes, nanosomes, ext.
I want to be very clear. Scientists are still working out the details on everything I just discussed regarding CRISPR delivery. This is a work in progress…Stay tuned.
What’s the latest in the world of CRISPR?
If you watched the TED talk video above, you heard Jennifer Doudna call for a moratorium on human embryonic CRISPR work.
That TED talk video is from Nov. 2015.
This moratorium never happened.
In February 2017, Beijing scientists published a report showing how they used CRISPR to make genome edits to a human embryo.
In the US, also in February 2017, the National Academy of Science released a statement. They essentially stated that they are comfortable with the idea of editing the DNA of human embryos (when serious disease is involved).
The science community regarded this NAS statement as a green light to modify human embryos in the United States.
In July 2017, a group of scientists from OHSU in Oregon, published this report demonstrating the efficacy of using CRISPR to modify human embryos. Shoukhrat Mitalipov, the lead scientist on this project, modified 22 embryos by injecting CRISPR at the 1-cell stage. He eliminated a mutation in a gene called MYBPC3. The mutated MYBPC3 is responsible for causing a myocardial disease. None of the modified human embryos from these publications were implanted into women. If they had been implanted, it is possible the embryos would develop into genetically modified babies.
Speaking of genetically modified babies…
In Novemeber 2018, a Chinese scientist named He Jiankui claimed to have created the world’s first genetically edited babies. He used CRISPR technology to introduce mutations into a gene called CCR5. In theory, if the CCR5 gene is mutated, then the HIV virus will be incapable of entering and destroying Helper T cells. If all goes perfectly, then He Jiankui will have created children that are immune to AIDS. Unfortunately, Jiankui went about this process with little regard for the twin girls in the experiment. He moved forward with the procedure even after he learned his CCR5 mutations had never been tested in animals. Animal testing in mice would at least determine whether a new CCR5 gene can lead to a toxic protein. He Jiankui’s approach to this project has led to widespread criticism from scientists, ethicists and government officials.
This begs the question…
Is it legal to genetically modify humans? Is it ethical? What’s the position of the US government on this issue?
What is the future of CRISPR? These are all good questions…We know the FDA is scrambling to keep up with this genome editing science. Occasionally, the FDA will place a clinical hold on a company’s plan to use CRISPR in a human clinical trial. These holds seems to be manageable and some companies are then given green lights to continue with modified plans.
The general public seems to be warming up to the idea of somatic mutations to eliminate a deadly disease.
Most importantly, it seems obvious that genome modification is marching forward regardless of the regulatory and ethical landscape.
The Future of CRISPR
Laboratory scientists are constantly innovating the design of CRISPR components. But which design will win out? To answer that question, we can look to ongoing human clinical trials. Clinical efficacy and safety are the only readouts that really matter. By that I mean, we need to apply CRISPR to human patients and then determine if this gene editing technology can effectively reduce symptoms of a disease while also remaining safe (i.e. not randomly mutating a patient’s DNA.)
As of 2020, we’re just beginning to see CRISPR technology move into human clinical trials.
Below, I list ongoing human clinical trials that rely on gene editing technology. I’ll update this table often to keep up with new trials.
Table 1. Human clinical trials based on gene editing technology (updated 2020)
Disease |
Group in pursuit (strategy) |
---|---|
Cancer (PD-1 knockout) | Hangzhou Cancer Hospital
Sichuan University
U Penn/Parker Institute |
Cancer (multiple myeloma) | CRISPR Therapeutics
|
Cancer (lymphoma) | CRISPR Therapeutics
|
Hemoglobinopathies (β-thalassemia, sickle cell disease) | Vertex Pharmaceutical/CRISPR Therapeutics |
Hemophilia B | Sangamo
|
HIV | Affiliated Hospital to Academy of Military Medical Sciences
Sangamo
|
Leber congenital amaurosis 10 (LCA10)
(hereditary blindness) |
Allergan/Editas |
MPS I (Hurler syndrome) | Sangamo
|
MPS II (Hunter’s syndrome) | Sangamo
|