The gene therapy sector is experiencing an acceleration.
The FDA, EMA and China are all approving gene therapy products for commercial use. In this article, I provide a brief background on the science and then discuss both the history and the future of gene therapy.
2021 update: I just updated our table of gene therapies in development. Click here to jump to our gene therapy pipeline.
author: Kevin Curran PhD
updated: 8-13-2021
For the past 50 years, the scientific community has been learning to modify human DNA using viruses. This work is extremely challenging and progress has been slow but steady. Scientists have struggled to design a gene therapy that can safely and effectively treat a disease.
In the most recent decade, we hit an inflection point.
In 2017, we saw two ex vivo, gene therapy drugs approved for the US market: Yescarta (Gilead) and Kymriah (Novartis). Now, in 2021, those first ex vivo gene therapies are routinely used to treat certain lymphoma cancers. Thousands of cancer patients have been treated with these genetic medicines.
Gene therapies for spinal muscular atrophy, inherited blindness, hemophilia, sickle cell disease and multiple myeloma are now used as clinical practice or else in late stage clinical trials.
In 2018, we witnessed a landmark FDA approval for a gene therapy product called Luxturna. Spark Therapeutics is using an AAV virus to deliver a gene that is missing in patients suffering from a rare, inherited eye disease. This treatment restores functional vision to the blind. Amazing! By 2020, over 200 vials of Luxturna have shipped out to patients.
In 2019, Novartis received FDA approval for a tragic condition called spinal muscular atrophy (SMA). Using an AAV9 viral vector, a gene therapy called Zolgensma delivers SMN protein into the motor neurons of afflicted patients. Zolgensma is injected into the patient’s blood then the AAV9 virus will target neurons and deliver the SMN gene into the DNA inside these neurons. The science is incredible and the price tag is equally incredible ($2.1 million per treatment). As of March 2021, Zolgensma has been approved in 38 countries and more than 1,000 patients have been treated with Zolgensma across clinical trials and in the commercial setting.

A successful gene therapy delivers a gene into the exact cells of our body that require this gene.
The commercially available gene therapies mentioned above are approved for rare diseases with small patient populations. The full impact of gene therapy will be reached when we see effective treatments for diseases with larger patient numbers (>200,000 patients). I am confident this will come to pass in the upcoming decade.
The FDA now expects to see a doubling of new gene therapy applications every year. Scott Gottlieb, the former FDA commissioner, predicted that by the year 2025, the US will be approving between 10 and 20 different gene therapies every year.
It can be a bit tricky to wrap your brain around this field. Most people I talk to (scientists and non-scientists) are not aware of the current state of gene therapy. Most people don’t know what has been done and what is coming down the pipeline.
I’ve created this page to bring people up to speed with the world of gene therapy. I’ll update the page every six months.
Here is a list of topics discussed below.
Click on a topic to jump straight to that section.
- Introduction to gene therapy (co-opting the virus, scientific challenges)
- History of gene therapy (1970-2010)
- Recent history: approved therapies (2010-2020)
- The future of gene therapy (pipeline 2021-2025, pricing challenges)
Click an icon below to share page with friends.
Introduction to gene therapy
Gene therapy is the use of genetic modification to treat human disease. A sick patient harbors a bad version of a gene, gene therapy delivers a good version of that gene into cells within the sick patient. The majority of diseases treated with gene therapy are inherited genetic diseases. However, you can also use gene therapy to treat certain cancers and viral infections.
The general strategy of gene therapy is relatively straightforward.
- Identify the genetic cause of a disease.
- In a laboratory, cut and paste some DNA so as to create a genetic fix for the problem.
- Design a delivery system to introduce this genetic fix into cells within the sick patient’s body.
Laying out this strategy on paper is simple, however, the reality of implementing a safe and effective gene therapy has been anything but easy.
The majority of clinical stage gene therapies employ a modified virus to deliver the genetic fix to either a human body or else to live human cells in a dish. Finessing this viral delivery system has been a challenge.
Before we delve into the history of this field of science, let’s examine the central role that the virus plays in gene therapy.
Sidenote: Gene therapy is different than genome editing. Gene therapy delivers a new gene into cells to compensate for a defective gene. However, gene therapy doesn’t remove or modify the defective DNA from a patients’ cells. In contrast, genome editing removes or modifies the defective DNA in its native location within the genome.
Importance of the virus in gene therapy
Before we think about a virus in the context of gene therapy, first let’s discuss the basics of naturally occurring viruses.
A virus is an infectious agent that can attach to the cells of our body. Viruses are parasites, they survive by infecting a host. Just like humans and bacteria, viruses evolved naturally here on earth.
Once attached to a human cell, the virus inserts its own genetic material (DNA or RNA) into the nucleus of the infected cells. This insertion allows the virus to replicate its own genetics within our cells. Once replicated, the virus moves on to infect nearby cells. Eventually, the host becomes sick from viral infection. Often, this infection leads to death.

Image: Thomas Splettstoesser, CC BY-SA 3.0,
A virus first attaches to the outside of a cell. The virus then enters the cell and injects virus DNA in the host cell DNA. The host cell then begins producing proteins from the virus DNA.
Interesting tidbit: A virus is not technically considered alive because a virus is not a cell-based organism. However, viruses do reproduce and contain genetic material and evolve through natural selection, so they do satisfy some criteria of life.
Semantics aside, viruses are dangerous and have been killing people since the dawn of humanity (HIV, Polio, Spanish flu, small pox, coronavirus)
In an interesting turn of fate, viruses are now being employed by the medical community as a means to save human lives.
In 1952, biologists first took notice of the efficient method a virus will use to inject DNA into the cells of their host.
In 1971, almost 20 years later, scientists began to use this viral injection method for the purpose of human gene therapy.
Today, the viral delivery deliver system remains the most common form of delivering genetic material via gene therapy.
Let’s examine how viruses are used in gene therapy…
The basic concept is simple…
- Identify the gene that is missing in a patient suffering from a genetic disease.
- Install this gene into the DNA of a modified virus. (A modified virus is no longer dangerous but can still infect cells.)
- Allow this modified virus to infect the cells of the sick patient.
- The virus inserts the missing human gene into the patients’ cells.
- These infected cells now contain a copy of the missing gene.
- Symptoms of the genetic disease begin to disappear.
- The disease is considered cured.

image: Simon Caulton, CC BY-SA 4.0
First identify the gene missing from a sick patient. Then head to the lab and create a modified virus that includes that missing gene.
Using a virus to conduct gene therapy is a classic example of scientists co-opting (i.e. hijacking) a natural biological phenomenon in order to create a biotech product.
Common forms of virus used for gene therapy.
Retrovirus
Many early gene therapy experiments used a type of virus called a retrovirus. A retrovirus infects cells with a single strand of RNA. Once inside a cell, this virus uses its own machinery (a reverse transcriptase enzyme) to produce DNA. This DNA then injects into the host cell genome. Many gene therapy trials rely on a modified lentivirus. A lentivirus is a type of retrovirus.
Adeno-associated virus
Recently, many gene therapy trials are conducted with a virus called an AAV (adeno-associated virus).
AAV is a small virus that infects humans and some monkeys.
AAV has become the preferred virus for the following reasons:
- only causes a mild immune response
- infects both dividing and non-dividing cells
- persists in cells without directly inserting into the host genome (remains in an extra-chromosomal state.)
One downside to AAV is that the carrying capacity of this viral vector is limited. This means it is not possible to insert large genes into an AAV. The size limit for AAV is debatable, but is generally listed between 4.5 to 4.9 kb. Scientists are currently trying to overcome this limitation.
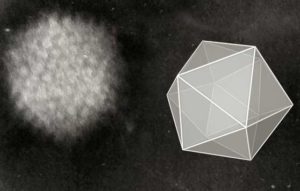
image: Graham Colm, CC BY-SA 3.0 from Wikimedia Commons
Electron micrograph of an adenovirus. Cartoon illustrates the icosahedral shape of the virus.
Challenges in the implementation of gene therapy
Scientist have been trying to insert missing genes into sick patients for about 40 years. Yet, we are just beginning to see the first FDA approvals. What took so long?
Well, science is always a long road…none of this is easy. Gene therapy also includes it’s own set of challenges and technical issues. I summarize these challenges below.
- Figuring out the best virus to use. I mentioned the virus needs to be modified before its used to deliver a gene safely. Its been tricky learning exactly how to make a virus safe and non-toxic for patients.
- Immune rejection. Our body is designed to reject and attack foreign DNA. Great lengths have been taken to reduce or eliminate any harmful immune response our body may generate in response to gene therapy.
- Creating a long term cure. Once the missing gene is inserted into our body, that gene may not stick around for long. Dividing cells may eventually stop reproducing the inserted gene. In that sense, the therapy is diluted out. This means the patient requires multiple treatments.
- Many diseases are caused by multiple genes. In fact, most inherited diseases are the cause of multiple genes. This complicates the gene delivery strategy. Delivering 1 gene is tricky… delivering 8 genes is very tricky.
- Ethical issues. Before moving forward with a therapy, you need to demonstrate that your proposed DNA modification is not germline (sperm or egg modification). This ensures we don’t alter the genetics of future humans, we only modify the sick patient.
- Inserting the gene in the right place. If your gene therapy strategy inserts the missing gene on top of another critical gene, then that critical gene becomes mutated and non-functional. CRISPR should help us direct a new gene into a precise location.
- High cost of therapy. The first gene therapy approved in Europe, named Glybera, arrived with a $1.6 million dollar price tag. As you may imagine, insurance companies were not thrilled to cover this price. Glybera was not utilized and is now regarded as a commercial failure. In recent news, Bluebird Bio has given up on selling their gene therapy in Europe. Zynteglo was approved in Europe for the treatment of a blood disorder called beta-thalassemia. Bluebird Bio set the price of this one-time treatment at $1.6M…. and European countries have refused to pay that price. Europe is sending a message to drug manufacturers that exorbitant drug prices won’t be tolerated. Drug companies insist their high cost reflects the long term benefits received by the patient.
European payers have not yet evolved their approach to gene therapy in a way that can recognize the innovation and the expected life-long benefit of these products.
~ Bluebird Bio President Andrew Obenshain (link)
History of gene therapy
“You have to know the past to understand the present.”
~ Carl Sagan
Gene therapy has been a work in progress for almost 40 years. Finally, in 2017, the US approved the first gene therapy treatments.
Q: How did we get to approved gene therapies?
A: Many brilliant and hard-working biologists have incrementally pushed this field forward.
Below, I summarize the significant milestones along the way.
The 1970s…
In 1971, a key scientific experiment revealed that DNA could be injected into human cells and that this injected DNA could fix a biological problem in those cells (Merril, 1971). The injected DNA restored enzyme activity that was missing from human fibroblast cells. These cells had been extracted from patients suffering from a disease called galactosemia. It is important to note that these cells were growing in a dish when they were treated with external DNA. In fact, it is much easier to manipulate the genetics of human cells once the cells have been removed from a human body.
Taking the next step… and delivering an effective gene therapy to a live human has been a major challenge throughout the past 40 years.
In 1972, a formal proposal to use gene therapy as a means to treat human genetic disease was first put forth in this Science article. Inspired by the 1971 results, the authors, Friedmann and Roblin, stated that ‘gene therapy may ameliorate some human genetic diseases in the future‘. The authors also expressed their hope that ‘gene therapy is used in humans only in those instances where it will prove beneficial‘ and will not be ‘misused through premature application.’
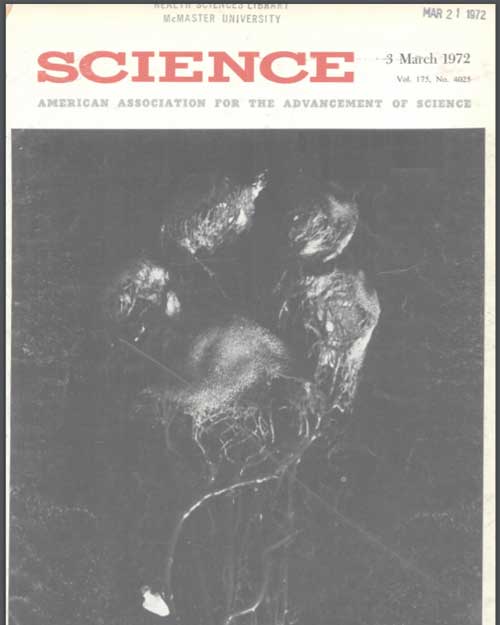
Gene therapy was first proposed in this 1972 Science journal.
The 1980s…
In the 1980s, scientists such as Martin Cline and French Anderson, were learning how to use viruses to deliver DNA into human or mouse cells. Initially these cells were genetically modified in vitro (growing in a dish outside a live human).
The first attempt to use gene therapy to treat live humans in clinical trials began in the late 1980s. These trials weren’t reported until the beginning of the 1990’s.
The 1990s…
In this 1990 report from NCI in Bethesda, scientists removed white blood cells from patients with advanced melanoma. In a laboratory, researchers modified the genetics of the white blood cells. A retrovirus was employed to insert a gene called interleukin-2. Finally, these genetically altered cells were infused back into the live patients. The report demonstrated that gene therapy could be delivered to live humans.
French Anderson performed a longer-term clinical trial to treat children with ADA-SCID disease. SCID stands for severe combined immuno-deficiency. The ADA refers to the fact that a specific gene, adenosine deaminase (ADA) is missing from these patients. Therefore, the goal for these clinical trials was the introduction of a healthy ADA gene into the ADA-SCID patients. A retrovirus was used to transfer the ADA gene into isolated T-cells. These gene-modified T cells were then infused back into the young patients. To everyone’s delight, a significant improvement in the health of the children was observed.
This report demonstrated that gene therapy could be safely and effectively administered to sick patients.
In a sad and disturbing footnote to this medical milestone, French Anderson was convicted of sexually abusing a minor in 2006. French spent 12 years in prison and was released on parole in 2018.
Gene therapy research continued in the 1990’s. Much of this work was focused on treating patients with ADA-SCID disease. Italian scientists successfully mixed stem cells and a retrovirus in order to deliver a full-sequence ADA gene into ADA-SCID patients.
Up until this point, both the NIH (National Institute of Health) and the FDA (Food and Drug Administration) were cautious but supportive of the development of this gene therapy work. However, that sentiment changed in 1999 when the first high-profile patient death occurred as a result of gene therapy. Jesse Gelsinger, an 18 year old, was born without a gene for ornithine trancarbamylase. A clinical trial run by the University of Pennsylvania delivered this missing gene into his body with an adenovirus.
4 days later, Jesse died as a result of a massive immune response that shut down multiple organs in his body.
This high profile death prompted the FDA to suspend several gene therapy clinical trials. The loss of Jesse Gelsinger was a wake up call that alerted people to the risks of this procedure.
2000-2020…approvals in Europe and United States
Gene therapy continues to march forward in this current decade. Notably, we have seen FDA approvals in the US and EMA approvals in Europe.
In 2012, Europe approved their first gene therapy treatment. The EMA (European Medicines Agency) approved Glybera, a viral treatment for a form of pancreatitis. This pancreas disease is caused by the absence of a gene called lipoprotein lipase. In 2015, the cost for treatment with Glybera was $1.2M Euros. This gave Glybera the infamous title of the ‘most expensive drug of 2015.’ It appears the gene therapy works on a biological level, but in a bizarre twist, the drug has been a complete failure. Payors refuse to pay for it. UniQure, the company that developed Glybera has already discontinued the drug after treating very few patients. As of 2018, Glybera is no longer listed on UniQure’s product pipeline.
This is a harsh lesson for the pharmaceutical industry. There is a limit to the amount you can charge for a drug…eventually insurance companies refuse to entertain your asking price.
In 2016, we saw another European approval for a gene therapy called Strimvelis. GalaxoSmithKline (GSK) developed Strimvelis, a stem cell gene therapy to treat ADA-SCID patients. ADA-SCID is a rare disease, affecting about 14 people per year in Europe. Similar to Glybera, this gene therapy got off to a bad start. The therapy was initially priced at $665,000. As of 2017, only 2 patients had received this treatment and GSK was attempting to sell off the therapy. That’s not a good sign. In 2018, Strimvelis was sold from GSK to Orchard Therapeutics. At point of sale, only 5 patients had received the product (price listed at $730K.)
Additional gene therapy trials are currently underway for:
- HIV (helper T-cells engineered with a CCR5 gene knock-out, ref.)
- Choroideremia (AAV virus used to insert REP1 gene, ref.)
- Sickle cell anemia (modified umbilical stem cell cord blood, ref.)
2017
In 2017, the United States finally approved its first gene therapy, a CAR-T. Similar to other therapies mentioned above, CAR-T involves removing blood from a patient, genetically re-engineering specific blood cells and finally infusing these genetically modified cells back into the sick patient’s body. In regards to CAR-T therapies, the T-cells (an immune cell) are injected with a new synthetic gene (a CAR). The CAR gene translates into a receptor protein that sits on the outside of the T-cell and helps the T-cell attack a specific type of cancer.
In August of 2017, the FDA approved the use of CAR-T therapy (Kymriah) for the treatment of ALL (acute lymphoblastic leukemia). Novartis designed this therapy. The T-cells are genetically modified to hunt down a marker (CD19) that is present on cancerous B-cells. B-cells are over-produced in ALL patients.
In October of 2017, the FDA approved the use of another CAR-T therapy (Yescarta) to treat adults with diffuse large B-cell lymphoma. This type of lymphoma is the most common form of NHL (non-Hodgkin lymphoma). Kite Pharma developed this therapy. Gilead acquired Kite Pharma in August 2017 for 11.9 billion dollars.
2018
Spark Therapeutics has been rewarded with an FDA approval for their Luxturna therapy. Spark uses an AAV vector to deliver a gene (RPE65) into the eye tissue of patients suffering from a rare disease called RPE65 mutation-associated retinal dystropy.
Sidenote: This Luxturna therapy is regarded as the first true gene therapy, because the viral treatment is delivered directly to the patient’s body. Previous gene therapy approvals (Yescarta and Kymriah) are called ex vivo treatments, as the cells receiving gene therapy (immune cells) are removed from the body prior to treatment.
2019
Avexis was awarded FDA approval for their gene therapy called zolgensma. Avexis is currently owned by Novartis. As mentioned earlier, this drug relies on an AAV9 viral vector that selectively targets motor neurons. The AAV9 virus injects a gene called SMN into the nucleus of a portion of the patient’s motor neurons. By analyzing an autopsy, scientists confirmed that the gene therapy was reaching the target cells. That is proof of principle that a IV injected AAV gene therapy can effectively reach neurons.
Recent developments in gene therapies in 2021
More gene therapies are expected to reach the market in the next few years. CAR-T approvals continue to pile up. CAR-T is an ex vivo, cell therapy involving the insertion of a gene into the patient’s immune cells. Recent CAR-T approvals include Tecartus (mantle cell lymphoma), Breyanzi (b-cell lymphomas) and Abecma (multiple myeloma).
Below I include a table that details gene therapies in various stages of development. I have updated this table on August 2021.
Okay – by now you should have a solid understanding of the history of gene therapy and how gene therapy works.
Now, I will share my thoughts on the future of gene therapy.
Future of gene therapy
The future is unwritten . . . but that won’t stop me from speculating.
Below, I present two topics relevant to the future of gene therapies. First, I provide a table of gene therapies in development. I then discuss a very contentious issue, the price of this healthcare product.
-
Gene Therapy Pipeline (list of treatments in clinic or on the market)
-
Price (get ready for sticker shock)
2021 Gene therapy pipeline
Therapy name | Company | Indication | Estimated U.S. patient population | Phase of development (projected U.S. launch) |
---|---|---|---|---|
Kymriah (tisagenlecleucel) | Novartis | B-cell precursor ALL, relapsed or refractory (r/r), large B-cell lymphoma | ~10,000 adult patients | FDA approved in 2017 |
Yescarta (axicabtagene ciloleucel) | Kite/Gilead | (r/r) large B-cell lymphoma | ~10,000 adult patients | FDA approved in 2017 |
Luxturna (voretigene neparvovec-rzyl) | Spark | RPE65 mutation-associated retinal dystrophy | 1,000-2,000 patients | FDA approved in 2017 |
Zolgensma (onasemnogene abeparvovec-xioi) | Jaanssen Pharmaceuticals/ Johnson & Johnson |
Spinal Muscular Atrophy (Type 1) | 8,000-10,000 adult patients |
FDA approved in 2019 |
Tecartus (brexucabtagene autoleucel) |
Gilead/Kite | (r/r) mantle cell lymphoma | ~1/200,000 | FDA approved in 2020 |
Abecma (idecabtagene vicleucel) | BMS/Celgene | (r/r) multiple myeloma | 9,000 adult patients | FDA approved in 2021 |
Breyanzi (lisocabtagene maraleucel) | BMS/Juno | (r/r) large B-cell lymphoma | ~10,000 adult patients | FDA approved in 2021 |
ciltacabtagene autoleucel |
Jaanssen Pharmaceuticals/ Johnson & Johnson |
(r/r) multiple myeloma in adults |
9,000 adult patients |
Phase III (2021) |
Tecartus (brexucabtagene autoleucel) |
Gilead/Kite | (r/r) B-precursor acute lymphoblastic leukemia in adults |
30,000 adult patients | Phase III (2021 ) |
Instiladrin (nadofaragene firadenovec) |
KD Therapies/ Ferring Pharmaceuticals |
(BCG)-refractory bladder cancer in adults; intravesical injection |
56,000 adult patients per year |
Pending FDA approval |
Kymriah (tisagenlecleucel) | Novartis | r/r follicular lymphoma |
86,000–100,000 adult patients |
Phase II (2022) |
Kymriah (tisagenlecleucel) | Novartis | 2nd line treatment of adults with r/r diffuse large B-cell lymphoma | 90,000 adult patients |
Phase III (2022) |
Yescarta (axicabtagene ciloleucel) | Kite/Gilead | 2nd line treatment of adults with r/r diffuse large B-cell lymphoma | 90,000 adult patients |
Phase III (2022) |
Breyanzi (lisocabtagene maraleucel) | BMS/Juno | 2nd line, r/r, aggressive, large B-cell lymphoma | 90,000 adult patients |
Phase III (2022) |
LentiD (elivaldogene autotemcel) |
Bluebird Bio | CALD in males aged less than 18 years | 700 pediatric patients |
Phase III (2022) |
eladocagene exuparvovec (fka AAVhAADC) |
Agilis Biotherapeutics/ PTC Therapeutics |
aromatic L-amino acid decarboxylase deficiency in pediatrics; intracerebral injection |
100 pediatric patients worldwide |
Phase II (2022) |
Zynteglo (betibeglogene autotemcel) |
Bluebird Bio | beta-thalassemia (subtypes) | 1,450 adult and pediatric patients |
Phase III (2022) |
beremagene geperpavec |
Krystal Biotech | dystrophic epidermolysis bullosa (DEB) |
900 adult and pediatric patients |
Phase III (2022) |
etranacogene dezaparvovec |
Uniqure | hemophilia B in adults | 1,800 adult patients |
Phase III (2022) |
Roctavian (valoctocogene roxaparvovec) |
BioMarin | severe hemophilia A in adults | 5,300 adult patients |
Phase III (2022) |
idanacogene elaparvovec |
Pfizer/ Spark Therapeutics |
hemophilia B in adults | 1,700 adult patients |
Phase III (2023) |
obecabtagene autoleucel (fka AUTO1) |
Autolus | acute lymphoblastic leukemia in adults |
30,000 adult patients |
Phase I/II (2023) |
RPL201 | Rocket Pharmaceuticals |
severe leukocyte adhesion deficiency type 1 (LAD-1) |
300 pediatric patients worldwide |
Phase I/II (2023) |
Engensis (donaperminogene seltoplasmid) |
Helixmith | diabetic peripheral neuropathy | 7.1–13.5 million adult patients |
Phase III (2023) |
debcoemagene autoficel |
Castle Creek Pharma | recessive dystrophic epidermolysis bullosa in patients aged 7 years and older |
400 adult and pediatric patient |
Phase III (2023) |
EB101 | Abeona Therapeutics |
RDEB in patients aged 6 years and older |
400 adult and pediatric patient |
Phase III (2023) |
Lumevoq (lenadogene nolparvovec) |
GenSight | Leber’s hereditary optic neuropathy, in adults with the ND4 mutation; ocular inj. |
4,500–7,500 adult patients |
Phase III (2023) |
olenasufligene relduparvovec (fka LYSSAF302) |
Lysogene/Sarepta Therapeutics |
mucopolysaccharidosis type IIIA, also called Sanfilippo Type A |
240–1,840 patients | Phase II/III (2023) |
PBCMA101 | Poseida Therapeutics |
(r/r) multiple myeloma |
9,000 adult patients | Phase II (2023) |
resamirigene bilparvovec |
Audentes Therapeutics |
X-linked myotubular myopathy in males aged younger than 5 years |
40 male newborns per year |
Phase I/II (2023) |
OTL103 | Orchard Therapeutics |
Wiskott Aldrich syndrome in pediatrics |
500 pediatric male patients |
Phase II (2023) |
atidarsagene autotemcel (fka OTL200) |
Orchard Therapeutics |
metachromatic leukodystrophy | 400–1,700 pediatric patients worldwide |
Phase II (2023) |
JNJ64400141 | Janssen Pharmaceuticals/ Johnson & Johnson |
respiratory syncytial virus-mediated lower respiratory tract disease in adults aged 60 years or older |
34 million adult patients |
Phase II (2023) |
LentiGlobin (beta-globin gene therapy) |
Bluebird Bio | sickle cell disease | 58,000 adult and pediatric patients |
Phase III (2023) |
fordadistrogene movaparvovec |
Pfizer | ambulatory patients with Duchenne muscular dystrophy |
4,000 pediatric males |
Phase III (2023) |
giroctocogene fitelparvovec |
Pfizer/Sangamo BioSciences |
severe hemophilia A in adults | 5,000 adult patients | Phase III (2023) |
ABO102 | Abeona Therapeutics |
mucopolysaccharidosis type IIIA, also called Sanfilippo Type A |
240–1,840 patients | Phase I/II (2024) |
ofranergene obadenovec |
VBL Therapeutics | recurrent platinum-resistant ovarian cancer |
15,000 patients aged 15 and older |
Phase III (2024) |
RPL102 | Rocket Pharmaceuticals |
Fanconi anemia in pediatrics | <1,000 pediatric patients |
Phase III (2024) |
Tavo (tavokinogene telsaplasmid) |
Merck/OncoSec | advanced or metastatic malignant melanoma in adults whose cancer has progressed on a checkpoint inhibitor |
subset of the ~1.2 million living with melanoma |
Phase III (2024) |
Zolgensma (onasemnogene abeparvovec-xioi) |
AveXis/ Novartis | spinal muscular atrophy Type 2 and Type 3 in pediatrics |
8,000 pediatric patients |
Phase I (2024) |
ProstAtak (aglatimagene besadenovec) |
Advantagene/ Candel Therapeutics |
intermediate to high risk, localized, prostate cancer |
125,000 adult patients |
Phase III (2024) |
Tecartus (brexucabtagene autoleucel) |
Gilead Sciences/ Kite |
r/r B-cell precursor acute lymphoblastic leukemia in pediatrics |
7,500 pediatric patients |
Phase I/II (2024) |
verbrinacogene setparvovec |
Freeline Therapeutics |
severe hemophilia B in adults | 1,700 adult patients | Phase I/II (2025) |
Invossa (tonogenchoncel-L) |
Kolon Group | knee osteoarthritis; intra-articular injection | 13 million adult patients |
Phase III (2025) |
nadofaragene firadenovec |
Trizell | r/r malignant pleural mesothelioma | 2,400 adult patients per year |
Phase III (2025) |
RGX314 | RegenxBio | neovascular (wet) age-related macular degeneration (AMD) |
2 million adult patients |
Phase III (2025) |
This table lists gene therapies in significant stages of the clinical pipeline. Many are in Phase 2 or 3, or else have demonstrated efficacy in Ph1/2 trials.
This pipeline changes quickly. I update every 3 months. Am I missing a significant development? Please let me know, you can contact me here.
Analyst reports and the CVS Health Clinical Affairs pipeline document was used as a reference in the table above.
If you’re interested in gene editing (CRISPR, ZFN, TALENS), I’ve also created this separate table of gene editing human clinical trials.
2021 update: I’ve been receiving a lot of email from people asking if a gene therapy trial is occurring for one particular disease. To help these folks, I’ve created a restricted search page on clinicaltrials.gov that only lists gene therapies. First click onto this restricted clinical trial search and then explore trials By Topic. Look for a disease in the topic list or else write in the disease on the adjacent search bar. This will provide a list of gene therapy clinical trials for one particular disease. If you see something of interest, click on a trial and you will find relevant contact information for that trial.
What is a fair price for gene therapy?
author: Kevin Curran PhD
updated: 8-15-2021
Zolgensma, a gene therapy for spinal muscular atrophy, was approved by the FDA in 2019. Novartis has priced this therapy at $2.1 million per patient. The science behind this new drug is ground-breaking. The value it brings to patients is also phenomenal. The cost of manufacturing this viral vector based therapy is not cheap. But, the question remains…does $2.1 M represent a fair price? Maybe…maybe not. Many folks in the healthcare industry are trying to make sense of these numbers.
First of all, when discussing gene therapy, people often use the word treatment, however if these therapies eliminate the medical condition, then by definition – we are talking about a cure.
If gene therapy delivers a cure…how much is that cure worth?
This is a challenging question that yields no quick answers. The healthcare industry doesn’t have a lot of experience delivering or pricing cures. Most drugs on the market offer treatments, not cures.
Organ transplants provide an interesting comparison as they’re considered a curative procedure. So… what’s the bill for a new organ? In the U.S. market, a heart transplant costs about 1.38 million, a liver is 812K and a new kidney runs 414K. Organ transplants aren’t cheap.
Are organ transplant prices indicative of the cost of future gene therapies?
Below, I’ll attempt to tackle this question by analyzing three different drug pricing paradigms. Before we get into that, let’s take a few items into consideration.
Much of the enormous price for gene therapies will be paid by either private insurance companies or Medicare. This means the general population (all of us) will ultimately share the tab via federal taxes or through higher premium rates/deductibles on our insurance policies. Therefore, we are all stakeholders in this decision.
That said, a drug company is free to price their gene therapy at any number they desire. Getting a drug approved by the FDA is incredibly difficult, although once the FDA approves a drug, there’s no government agency that regulates the price.
Drug companies often price their drug based on the dollar amount they think they can get from the market.
The problem is…supply and demand economics is a blunt and ugly tool to use when considering life and death situations.
In this regard, healthcare is fundamentally different than other industries.
A car company may price a Mercedes Benz at 60K … and that’s fine. If you don’t care to spend 60K on a car, then walk around the corner and test drive a Honda Civic. In contrast, if your child is born with a life-threatening genetic disorder, then you (or your insurance company) will be compelled/obligated to pay any price to save that human life.
The element of consumer choice is severely reduced in healthcare decisions. If a drug company is free to charge exorbitant costs for a life-saving treatment, the patient will justifiably feel that their life is held at ransom. Nobody wants that. Nobody wants a drug’s price tag to take on the tone of a ransom note.
Yet, at the same time…drug manufacturers must be incentivized.
Breakthroughs in science come at a high cost. Pushing a drug through the FDA pipeline is astronomically expensive. One report estimates an average cost of 2.5 billion to finance the FDA approval of a single drug. Innovators deserve a reward if they develop a successful gene therapy.
So, where does this leave us?
Is there a reasonable process to use when determining a fair price for an effective gene therapy?
Below, I examine this question from three different paradigms.
- Determine the cost of not treating the genetic disorder. Price a gene therapy under that number.
- Price a gene therapy as competitive to a preexisting treatment for the same disease.
- Use a cost-effective ratio to set price based on the number of quality-adjusted life years a therapy delivers.
Before I continue, I will again point out that drug companies are free to price their drug wherever they want. However, the payors bat last. Payors reserve the right to deny reimbursement for the drug. For about 90% of Americans, Medicare and private insurance companies pay for the majority of drug costs. If a gene therapy company is too ambitious with their price-tag, it is possible a payor will deny coverage and instruct a hospital or a physician to prescribe the next best drug on the list of options. To underscore this point, in August of 2021, Bluebird Bio announced they were giving up on delivering their gene therapy to European markets. The reason for their retreat?….European payors refused to pay the $1.8M price-tag for Bluebird Bio’s gene therapy for beta-thalassemia.
Determine the cost of not treating the genetic disorder. Price your gene therapy under that number.
Luxturna is the first true gene therapy product approved in the United States. This therapy treats an inherited form of blindness called RPE65 Mutation-associated Retinal Dystrophy. Luxturna is regarded as a cure for blindness. Blind people are regaining functional vision so that they can lead independent lives.
In 41 patients in the clinical program, a single dose of Luxturna restored functional vision in these patients—and in a way that they were now able to conduct activities of daily living independently. The latest data, presented at the American Academy of Ophthalmology, suggests that one dose at three years and counting is still showing a sustained effect.
As the quote above communicates, the trial patients seem to be doing well. A recent report confirmed that improvements in vision were maintained four years after administration.
Luxturna is now in the commercial phase and Spark is starting to see revenue on their product.
Spark is selling Luxturna for 425K per eye, so 850K for both eyes. Luxturna was approved in late 2017. As of 2020, about 200 vials of Luxturna gene therapy had been shipped to patients. 200 vials shipped is a relatively small number, but keep in mind, this is a rare disease and not all patients are eligible for the treatment.
Other rare disease gene therapy companies are looking to the rollout of Luxturna and Zolgensma as a guide to help them set their pricing strategy. Many factors must be considered when setting the price of a drug. For Spark, one of these factors is the cost of the alternative option. In the case of blindness (RPE65 Mutation-associated Retinal Dystrophy), there is no alternative treatment.
Therefore, when explaining the price of Luxturna, Jeff Marrazzo (CEO of Spark) compares his drug price against the cost of an untreated, blind child.
“When you do that pharma-economic modeling exercise, what we saw in evaluating it was that the worth of that was in excess of a million dollars,” said Marrazzo. “When you actually put together the cost of educating a blind child, the cost of loss in productivity for someone who has to care for a blind child, or productivity losses in terms of themselves. That’s what it amounts to—and this was a great set of out-of-the-box thinking by our commercial team.”
In summary, the CEO of Spark argues that an untreated blind child will require more than 1 million in expenses throughout the course of their life. In comparison, his gene therapy will restore their functional vision and only cost 850K.
That seems like a total saving of 250K!
What’s not to like??
Well, what happens if the gene therapy only restores 50% vision functionality to the patient? That treated patient may still require medical assistance in the years ahead. If that’s the case, shouldn’t the cost of the gene therapy be 50% of the cost of an untreated child?
We also need to consider the reimbursement timeframe. An untreated blind child will require assistance incrementally throughout their life. Their medical and educational costs would be spread across a range of payors over the course of decades. In the case of a single dose treatment, the gene therapy manufacturer would like their full price paid up front in one lump sum. In this sense, a genetic fix front loads all of the potential costs of the disorder into one bill. Insurance companies don’t love this.
Price your gene therapy as competitive to a preexisting treatment for the same disease.
In the case of hemophilia A and spinal muscular atrophy (SMA), we do have alternative drugs on the market. SMA can be treated with Spinraza and hemophilia A is currently treated with factor VIII replacement therapies. As gene therapy companies contemplate pricing strategies, their executives will be comparing the price for a one-time, gene therapy treatment vs. the total cost, year over year, for the preexisting treatment.
In the case of hemophilia A, analysts predict a gene therapy cure could be marketed in the U.S. and Europe for as much as $3 million.
About 20,000 Americans have hemophilia A. Analysts estimate that factor replacement therapy, the current treatment for hemophiliacs, costs between $580K and $800K per year for adult patients. For some patients, total annual costs can approach $1 million.
Therefore, a potential one-time $3 million cost for a gene therapy cure would represent an enormous bargain compared with a lifetime of chronic therapy with factor replacement therapy.
A similar discussion is currently happening in the context of SMA. Novartis spent $8.7 billion to purchase AveXis, a gene therapy company working on a cure for SMA. Zolgensma, the name of this gene therapy product, was approved in 2019. Novartis has priced this therapy at $2.1 million. They argue that a $2.1 million price tag is still much cheaper than the standard of care, Spinraza.
Spinraza, an RNA splice modifier drug, is the preexisting treatment for SMA. Biogen owns Spinraza and they charge 750K for the first year of treatment and 375K for each subsequent year of treatment. Therefore, by pricing their gene therapy at 2.1 million, Novartis can still make the case that they’re a bargain compared to a lifetime (or even 5 years) of Spinraza treatment.
The math works out …. but again, the missing piece here is the extent of the health benefit. Does the genetic fix offer superior health benefits and lower side effects than the pre-existing treatment?
The next pricing paradigm takes the quality of health benefits into consideration.
Use a cost-effective approach for pricing
The cost-effectiveness ratio compares the drug price to the drug’s medical benefit.
Ideally, the cost of a gene therapy will only be high when the delivered health benefit is also high. Reciprocally, if a gene therapy delivers meager health benefits, then the price should be low. Therefore, an appropriate drug price will reflect a 1:1 cost-effective ratio. That is the essence of a cost-effective approach to drug pricing.
Ok, so how do we arrive at the numbers for this ratio.
Cost is fairly straightforward, it’s the price of the drug.
The health benefit of a drug is more difficult to quantify. To approach the right number, the industry relies on a metric called a QALY.
QALY is an acronym for quality-adjusted life year.
QALY calculation
We first assume that health is a function of length of life and quality of life. A QALY score combines these values into a single number.
To determine QALYs, you multiply the value associated with a given state of health by the years lived in that state.
A year of life lived in perfect health is worth 1 QALY. (1.0 year of life × 1.0 health value = 1 QALY)
Now, let’s imagine you have a genetic disorder and you want to compare the QALY of 2 different treatments for that disorder.
This short and sweet video does a great job describing QALYs.
Treatment A delivers 8 years of life lived in perfect health. (8 years of life x 1.0 health value = 8 QALY)
Treatment B delivers 13 years of life but those years are not perfect. Most patients experience extreme nausea and require long hospital visits. The health value of these years is 0.5. (13 years of life x 0.5 health value = 6.5 QALY)
In terms of QALY score, Treatment A (8) is a better choice than Treatment B (6.5).
Placing a dollar amount on 1 QALY
Guiding principles underlying cost-effectiveness analysis:
- There’s not enough insurance money to finance every possible treatment.
- The stakeholders (insurance, hospitals, tax-payers) want to deliver the best medical outcome per dollar.
- Treatments that deliver many quality life years for a reasonable cost should be preferentially funded.
Suppose a drug adds one year to your life. What is the monetary value of one life year?
Is it possible to place a dollar value on one year of being alive? In reality, the answer is no. Life is a personal, spiritual and abstract concept. Life can’t be reduced to a column on an excel spreadsheet. But, for the sake of this discussion, let’s say….yes, life can reduce down to a value on a spreadsheet. The average value of one human life year is worth $50,000.
50K is the life year value that has been used historically by many private and government-run health insurance plans worldwide. Recently, other groups assign a larger value (100-150K per QALY).
By introducing a dollar amount to a QALY, we can now compare the cost of the drug with the drug’s medical benefit.
If a drug delivers one extra year of perfect health, then the drug should cost 50K.
1 QALY = 50K
If the drug delivers one extra year but that year includes excessive nausea and long hospital visits, then the drug should cost 25K.
0.5 QALY = 25K
Thinking about QALYs in the context of gene therapy…
Let’s say you suffer from a genetic disorder and Drug X can add 10 half-quality years to your life and you need to take a pill every month for the benefit.
That’s 10 years x 0.5 health value = 5 QALY.
5 QALY at 50K/QALY = 250K.
Therefore, a total fair price for Drug X is 250K as this would represent a 1:1 cost-effective ratio.
How would Drug X compare to a gene therapy cure for the same disease. In theory, a gene therapy will require a one-time payment for a one-time administration of a viral vector that eliminates the symptoms and the source of a genetic disorder.
How many QALYs is that cure worth?
If a gene therapy introduces perfect health to the patient, then these extra years have a health value of 1.0.
If the gene therapy is administered to a 10 year old child, then how many healthy years are delivered?
For the sake of this best-case scenario, let’s say the benefits of the gene therapy do not diminish over time. We assume the treated child will go on to lead a full life. Average life expectancy in the U.S. is 78. If the child is treated at 10 and lives in great health till 78, then how many QALYs is that?
78-10=68 years
68 years x 1.0 health value = 68 QALY
68 QALY x 50K/QALY = 3.4 million dollars for the price of the gene therapy.
3.4M is a large number. It’s also a number that insurance companies and Medicare may not like.
However, a lot of generous assumptions went into that equation. I assumed the gene therapy is administered to a young child and I assumed the therapy delivers a full life with zero quality of life issues. How will the equation change when eligible patients are spread across a broad age range? How will the equation change if the therapy must be re-administered every 3 years. Each administration could involve some degree of liver damage, steroid treatment and a hospital stay. Does that diminish the QALY score? The burden of proof is on the drug company to demonstrate the long-term quality of medical benefit their gene therapy delivers.
As you can tell, I don’t pretend to have an easy solution to the issue of fair price for gene therapy.
That said, I am in favor of using a cost-effective approach. QALY scores provide a reasonable platform for the analysis of gene therapy and price. These metrics keep the focus of appropriate drug price centered on patient experience and the quality of medical outcome. I think that’s the right place to ground the conversation.